Growing an eye for transplantation: potentials and pitfalls
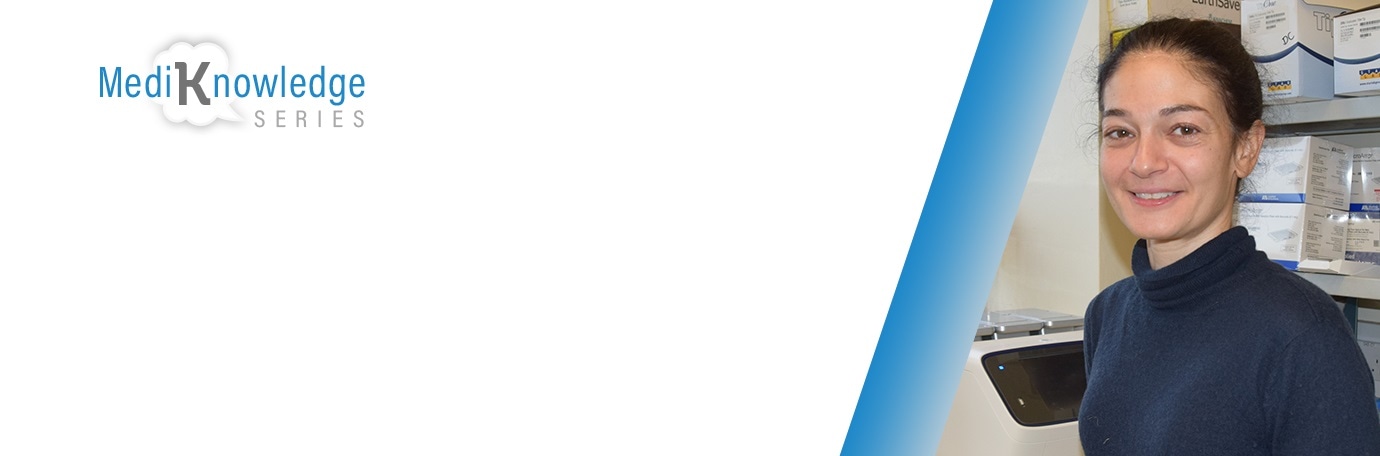
Neuro-ophthalmology at the Bristol Eye Hospital
Scientists have been working hard to bring about a time in the future when we can grow “an eye in a dish” and use it for transplantation to restore the vision of someone who is blind, and they are not as far off as you might think.
It is important to understand how eye development normally happens so that we can try to replicate it in the lab. We have identified a number of important genes that have a major impact on eye development when they are faulty.
For example, some genetic mutations lead to anophthalmia (one or no eyes present) or microphthalmia (abnormally small eyes) whereas others lead to cell-specific defects like the absence of rods or cones at the back of the eye, the cells (photoreceptors) that are responsible for detecting light.
T2-weighted MR scan of a patient with unilateral anophthalmia. Note the presence of amorphous tissue and structures resembling extraocular muscles within the anophthalmic right orbit. The right optic nerve/chiasm junction appears attenuated rather than absent suggesting possible residual optic nerve neural tissue. © Verma AS / Wikimedia Commons.
Growing an eye in the lab
The genes that determine where an eye develops, how large it will be, the cell types that develop within it and the function of those cells is very tightly regulated, as you might imagine. The key is to switch them on in the right place at the right time and in the right order. Regulatory proteins called transcription factors control this.
Transcription factors are like the conductor in an orchestra, signalling which musicians need to play during each bar of a symphony. Like the conductor in an orchestra, transcription factors regulate which of many thousands of genes need to “play” during each stage during the development of the human embryo and eye.
Transcription factors and the genes they regulate are all coded in our DNA. DNA is essentially the instruction manual of a developing human that is packaged into the single cell that forms when sperm fertilises egg.
This cell divides into two cells, then four, then eight, until a ball of cells is formed which each have the potential to form an entire human, placenta and amniotic cavity following the instructions in our DNA. These cells are truly omnipotent!
A cavity forms inside the egg, and the embryo develops from a collection of cells inside the cavity called the inner cell mass.
As these cells are limited to growing into an embryo (not placenta or amniotic sac), they are called pluripotent rather than omnipotent. When scientists refer to human embryonic stem cells (hESCs), they mean the pluripotent cells of the inner cell mass.
A major scientific discovery was that hESCs could be cultured in the lab to grow into 3D eye-like structures, simply by adding certain nutrients and growth factors into the culture media. It appears that once the hESCs had been set on this developmental pathway, they were able to organise themselves into forming an eye, without the forced expression of any transcription factors.
The drawback is that hESCs are taken from embryos left over following IVF treatment. Their use is tightly regulated and the ethics of using this tissue for research are hotly debated.
A further breakthrough occurred when scientists found that fully differentiated cells, e.g. skin cells, could be reprogrammed to go back in time and become “induced” pluripotent stem cells (iPSCs). All this seems to require is the forced expression of four transcription factors in skin cells that are normally only expressed in stem cells.
This discovery opened up the possibility of taking a skin biopsy from a patient and reprogramming the cells back into iPSCs, so that they could be grown into eye tissues in the lab, using the patient’s own cells rather than hESCs.
Future challenges
There are still many challenges to address before we can use eyes grown in the lab for transplantation. The retina has a complicated circuitry comprised of nerve cells that are important for processing visual information into a perceived image.
It is not so simple to transplant an eye or individual cells taken from one eye and place them in another, expecting them to connect up perfectly with all the nerves responsible for normal vision.
For example, there are physical barriers that transplanted rods and cones would have to overcome to position themselves in the right place and connect with nerves in the host retina.
The disease processes that lead to blindness in the first place may have led to scarring and remodelling of the host retina, so that transplanted cells have nothing left to connect with.
It is easier to transplant other cell types in the eye that do not have these issues, like retinal pigment epithelial cells (RPE) which can also be grown from hESCs and iPSCs in the lab.
RPE cells are positioned next to the rod and cone photoreceptors, but they are not involved in the complicated circuitry of the retina. Their role is to supply nutrients to the photoreceptors, take away waste products, and recycle light sensitive molecules.
Some common causes of blindness, like age-related macular degeneration, are actually diseases of the RPE – photoreceptors get damaged as bystanders to the inflammation and bleeding that occur.
That is why clinical trials testing the efficacy of stem cell therapies are transplanting RPE cells and not photoreceptors. So far, the results seem promising and the transplantation of RPE cells appears to be safe.
So the time in the future when we can grow “an eye in a dish” and use it for transplantation to restore the vision of someone who is blind, is not so very far away.
Acknowledgements
Fight for sight – Someone in the world goes blind every five seconds.
Our mission is to stop sight loss in its tracks. By funding pioneering eye research, we’re creating a future everyone can see.
Further Reading
- All Eye Surgery Content
- Small Incision Lenticule Extraction (SMILE) Surgery
- LASIK – What is LASIK?
- LASIK Complications
- Oculoplastic Surgery Overview
About Dr Denize Atan
Dr Atan completed her medical training at the University of Cambridge then Oxford.
After specialising in general medicine and obtaining Membership of the Royal college of Physicians (MRCP, UK), she became increasingly interested in the great variety of systemic diseases that affect the eye and vision in general.
She undertook her specialist training in ophthalmology in the South West region, including the Oxford Eye Hospital and Bristol Eye Hospital, and it was in Bristol that her current research interest in ocular genetics was ignited.
Dr Atan completed a PhD in ocular genetics from the University of Bristol in 2008, followed by a Wellcome Trust funded post-doctoral research fellowship in Toronto, Canada. She finished her training in ophthalmology and received the Harcourt Medal for the highest mark in the Fellowship exams of the Royal College of Ophthalmologists (FRCOphth).
She later developed her clinical expertise in Neuro-Ophthalmology with a Senior Fellowship in Birmingham and currently works as Senior Clinical Lecturer and Honorary Consultant in Neuro-ophthalmology at the Bristol Eye Hospital.
Dr Atan actively promotes public engagement with science, equality for men and women in science and the need for vision research.
Disclaimer: This article has not been subjected to peer review and is presented as the personal views of a qualified expert in the subject in accordance with the general terms and condition of use of the News-Medical.Net website.
Last Updated: Jun 25, 2019
Source: Read Full Article